Plinian eruptions, also known as Vesuvian eruptions, are the largest, most explosive, powerful, and catastrophic of all volcanic eruptions. They are characterized by sustained eruption columns reaching the stratosphere, tens of miles high, with a mushroom-like peak.
These eruption types are named after Pliny the Younger, who wrote an in-depth account of the 79 AD Mount Vesuvius eruption. Their other name, Vesuvian, is from Mt. Vesuvius in Italy, after which these eruptions are modeled.
Pliny the Younger, who observed the eruption from a town across the bay, described it as of an unusual size and shape and compared it to a pine tree with an extremely long trunk. He noted that at its top, its shape resembled pine branches spreading outward.
In his description, Pliny also mentioned that the eruption appeared bright, sometimes spotted, dark, and contained what looked like cinder and earth.
Lastly, the 79 AD Mt. Vesuvius eruption killed about 2000 people, including Pliny the Elder. Pliny the Elder probably died from suffocation or from inhaling poisonous gases as he attempted to rescue his friends and family.
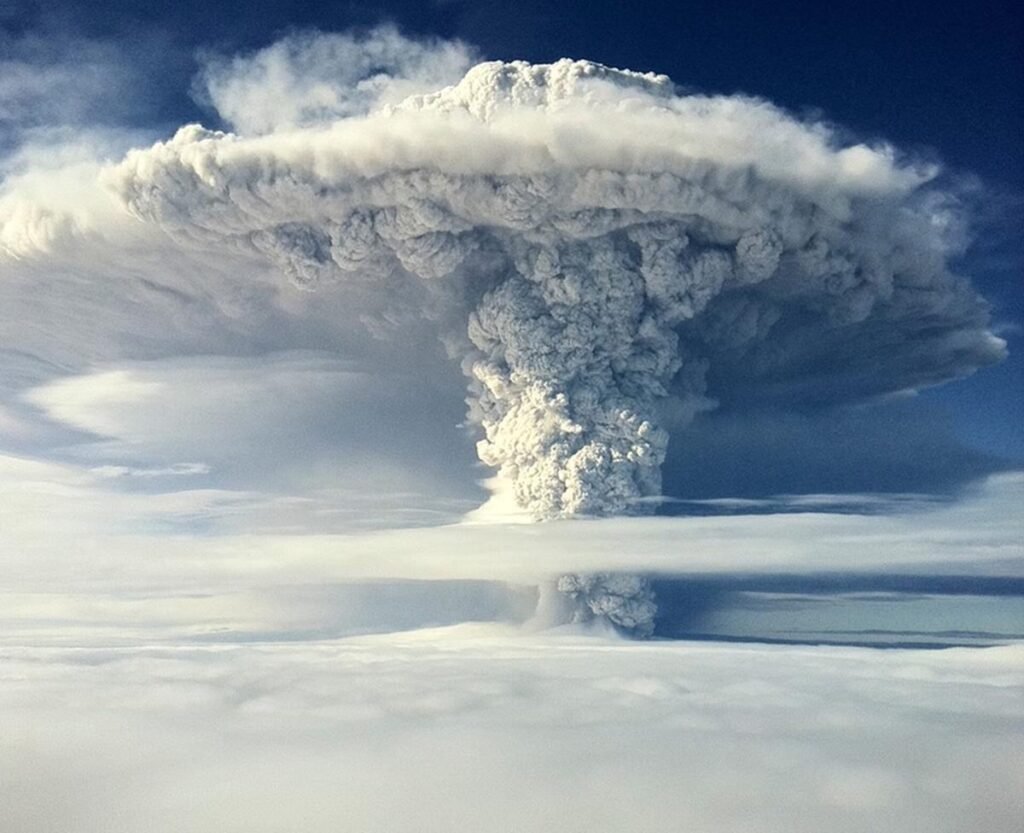
Characteristics of Vesuvian or Plinian eruptions
Some of the characteristics of Plinian eruptions include the following:
1. Largest, most explosive and with high discharge rates
Plinian eruptions are the most powerful, explosive, and destructive of all volcanic eruptions. They have the highest discharge rates, speeds, volumes, and volcanic explosivity index (VEI).
Their volcanic explosivity index is 5-8, discharge speeds are up to hundreds of meters per second, and discharge rates are in the order of 106-108 kg/s.
2. Huge volume of pyroclasts distributed over large areas
Plinian eruptions discharge a large volume of magma, usually tens of cubic kilometers, and the resulting tephra will cover extensive areas of up to 50,000 km2 downwind, especially ashfalls.
Fine ash and aerosols ejected during these eruptions can encircle the earth’s atmosphere within a few weeks. These ashes and aerosols may also cause a global red sunset appearance, with some aerosols remaining in the atmosphere for years.
Vesuvian eruptions will eject a mixture of gases and pyroclasts made of ash, lapilli, bombs, and blocks, which can be lithic, juvenile, or cognate fragments. Volcanic ash and pumice lapilli usually dominate the pyroclasts, and most of the juvenile fragments are highly vesicular.
3. Sustained, tall column reaching stratosphere
Plinian eruptions have nearly continuous or sustained, tall, often dark eruption columns of pyroclasts and gases. These eruptive columns reach the stratosphere, which is 10-50 km (7.5-31 mi) above the earth’s surface and have mushroom- or umbrella-shaped peaks.
These columns resemble huge rocks going up to the atmosphere from the launch pad or an atomic bomb explosion.
4. Have Lightning strikes and loud noises
Vesuvian eruptions will produce loud, thunderous noises and lightning strikes. As the produced volcanic plume rises, particle collisions and friction create static electric charges that will accumulate in the air. The sudden release of these static electric charges causes lighting strikes.
5. Associated with PDCs
Although these columns are nearly continuous or sustained, they pulse with changes in eruption intensity or may sometimes collapse. The collapse of eruptive columns often triggers pyroclastic density currents (PDCs).
6. Involve volatile rich, mostly silicic magmas
Plinian or Vesuvian eruptions involve mostly volatile and silica-rich, highly viscous felsic magmas. Some may, however, form from intermediate magmas like andesite and less often mafic magmas such as basalt.
7. Are most thermally efficient
These eruptions produce highly fragmented pyroclasts, meaning they effectively convert thermal energy into mechanical energy that breaks down the fragments.
Magma composition
Most of the Plinian eruptions form from felsic magmas rich in volatiles like rhyolite, dacite, rhyodacite, phonolite, and trachyte. These magmas have more than 63% silica. However, these eruptions can occur in intermediate magmas with 52–63% silica, like andesite and trachyandesite, and less often from mafic or basaltic magmas with 45–52% silica.
Notable examples of volcanoes that erupted silica-rich or felsic magmas are Vesuvius Italy (79 AD), Krakatoa in Indonesia (1883), Novarupta in Alaska USA (1912), and Chaitén in Chile (2008).
Plinian eruptions with intermediate magma composition include Hekla in Iceland (1947), Volcán de Colima in México (1913), Mt. Tarumae in Japan (1667 and 1739), and Ruapehu New Zealand (27-10 ka BP).
Although uncommon, basaltic eruptions like Mount Tarawera in New Zealand (1886), Mt. Etna in Italy (122 BC), and Las Sierras-Masaya in Nicaragua (1670 and 1772) are excellent Plinian examples.
Most of the Plinian eruptions that involve basaltic magmas have silica-rich top chambers. These chambers with highly differentiated magmas are what drive the initial, explosive eruption phase.
Finally, some eruptions can have more than one type of magma. For instance, on May 18, 1980, Mt. St. Hellen in Washington, USA, erupted rhyolite, dacite, and andesite. Similarly, the 1707–1708 Hōei eruption in Japan had dacite, andesite, and basalt magmas.
Vesuvian or Plinian eruption formation
Plinian eruptions form when volatile-rich magma rises towards the earth’s surface. As magma rises, a drop in pressure will cause degassing, i.e., volatiles will exsolve, forming gas bubbles.
Plinian eruptions form when volatile-rich magma rises towards the earth’s surface. As magma rises, pressure drops will cause degassing. Degassing occurs when volatiles exsolve, forming gas bubbles.
The high viscosity prevents the formed gas bubbles from rising faster than the rest of the magma body. While they don’t rise faster or escape, these gas bubbles increase the magma buoyancy and accelerate it.
When the rising magma approaches the earth’s surface, the rapidly expanding gas bubbles will violently tear and blast magma clots, lithic rocks, or anything on their path into the air. The grinding and milling that occurs between ejected fragments will also reduce particle sizes.
1. Eruptive column formation
The ejected gas and pyroclasts will form a nearly sustained eruptive column due to the supply of high volumes of volatile, rich magma.
This eruptive column has a jet phase near the vent, a tall convective phase in the middle, and a mushroom- or umbrella-shaped phase at the peak.
The jet phase will have high velocities of 100-600 m/s and cover the first hundreds to thousands of meters.
As the jet phase rises, it will incorporate more air. This will lower its bulk density compared to surrounding air, creating a convective phase. The convective phase moves at modest speeds and represents the tallest section of the eruptive column.
When the bulk density of the convective phase equals that of surrounding air, the eruptive column will start quickly spreading laterally, forming an umbrella-shaped cloud. This ash cloud will spread fast, covering large areas within a short time, and it will also result in extensive ash falls.
2. Eruptive column collapse
A decrease in magma supply, lower discharge rates, or a reduction in volatiles can reduce the eruptive column’s buoyancy, causing it to collapse. Lower magma discharge speeds and an increase in the vent size can also cause the collapse of the eruptive column.
The collapse of eruptive columns will cause pyroclastic density currents (PDCs). PDCs form commonly when the magma has high discharge rates with the existing velocity. However, the vent radius can also influence the formation of PDCs.
Besides PDCs, Plinian eruptions can also form domes, lahars, calderas, or lava flows. Lava domes form if magma degassing occurs or gases leak. The May 1980 eruption at Mt. Helens in Washington State, USA, formed a lava dome.
If magma degasses, it will form thick, slow-moving lava flows. Most lava flows form towards the end of Plinian eruptions.
Plinian eruption deposits and landforms
Plinian eruptions will deposit huge amounts of tephra, especially pumice and ash. However, they will also deposit lapilli, cinders, volcanic bombs, and blocks.
Pumice and ash can travel over 250 miles (402 km) downwind, while larger pyroclasts like bombs, blocks, or lapilli will fall closer to the vent.
Common landforms associated with these eruptions include ignimbrite, ashfalls, pumice, and ash flow deposits.
Deposits from ashfall or tephra are well sorted, while those from PCDs will have poorly sorted pumice, lapilli, and ignimbrite.
Sub-Plinian and ultra-Plinian
Depending on their intensity, these eruptions fall into sub-Plinian, Plinian, or ultra-Plinian categories.
Sub-Plinian are less explosive, have lower discharge rates, shorter eruptive columns, and disperse tephra to a relatively smaller area. Their eruptive columns go 10-20 km or up to 30 km above the earth’s surface, and discharge rates are 106-107 kg/s.
Sub-Plinian eruptions will produce sustained but short-lived eruptive columns, lasting a few minutes to hours. These columns may pulse, and strong winds will bend them.
Sub-Plinian eruptions will deposit fine or coarse-grained, thin beds of tephra. The deposited tephra will widely vary in vesicularity, clast morphology, and vesicle shapes when compared to ultra-Plinian tephra.
The common Sub-Plinian eruption examples include the Mt. Redoubt (1989-1990) and the Mt. Spurr (1992), both in Alaska, USA. Mount St. Helens in Washington, USA, also erupted six months after the May 18, 1980, eruption.
On the other hand, ultra-Plinian eruptions are the most powerful of all volcanic eruptions. They have the highest discharge rates, discharge the largest magma volume, and have the tallest eruptive columns, reaching up to 55 km into the atmosphere. Also, their tephra covers larger areas.
Examples of ultra-Plinian eruptions are the Tambora in Indonesia (1812-1815) and the Taupo in New Zealand (180 AD).
Lastly, eruptions that eject over 100 cubic miles of tephra are known as supervolcanoes.
Notable examples of Vesuvian or Plinian eruptions
Some of the notable sub-Plinian, Plinian, and Ultra-Plinian eruptions in the US include the Long Valley Caldera in California (760,000 PB), Katmai (June 10, 1902), Mount Spurr (June 27, 1992), Alaska, and Mt. St. Helens in Washington (May 18, 1980).
Examples of Plinian eruptions in other countries are Mt. Etna (122 BC) and Mt. Vesuvius (AD 79) in Italy, Mt. Toba (74,000 BP) in Indonesia, Minoan (1600 BC) in Santorini, Greece, and Nevado del Ruiz (November 13, 1595) in Colombia.
More examples include Krakatoa (1883) and Tambora (April 5, 1815) in Indonesia, Mt. Pinatubo (June 15, 1991) in the Philippines, Chaitén (May 2008) in Chile, and Volcán de Colima (1913) in México, and Hekla (1947) in Iceland.
Finally, some of the Plinian eruptions in New Zealand are Tarawera (June 10, 1886), Taupo (AD 232), and Hatepe (AD 232).
What are the hazards of Plinian eruptions?
Plinian eruptions and associated hazards like lahars, pyroclastic density currents, or the emission of poisonous gases can kill people and animals. These eruptions will also destroy, bury, or incinerate towns, villages, vegetation, and agricultural lands.
Additionally, the ejected ashes and aerosols can cause hazards to the aviation industry and may affect the global climate. Aerosols from these eruptions can, for instance, block the sun in the atmosphere, causing a slight drop in temperature.
Furthermore, the 1915 Mount Tambora eruption resulted in a year (1916) without summer in northeastern USA, Canada, and Europe.
Frequently asked questions
How explosive a Plinian eruption is will depend on volatile content, the number of nucleation sites where bubbles form, the volume of the erupting magma, and the radius of the vent. The exit pressure and how quickly magma rises or decompresses will also affect the explosiveness of these eruptions.
They are common above subduction plate margins, with a few known to occur in volcanic ocean ridges.
Ash beds from eruptions act as isochronous marker beds for correlation since they are deposited instantaneously over large areas. By studying the deposition characteristics, geologists can also tell about past events such as the wind direction and strength.
Most Plinian eruptions erupt at 750–1000 °C (1382–1832 °F). However, temperature will depend on the kind of magma involved. Basaltic and intermediate magmas erupt at higher temperatures than felsic.